Measuring Dk Temperature Changes in PCB Circuit Materials
Temperature characterization parameters of PCB circuit materials can provide insight into how temperature variations in the operating environment affect mmWave and high-speed digital (HSD) circuit performance.
Material operating temperature is an often overlooked circuit material parameter. However, elevated operating temperatures caused by heat sources inside or outside the board can cause changes in circuit performance, especially at millimeter-wave frequencies and in high-speed circuits. By studying key temperature-dependent material parameters of circuit materials, such as the thermal coefficient of dielectric constant (TCDk) and the temperature coefficient of insertion loss (TCIL), the temperature-dependent performance of high-frequency circuit materials can be better understood. Comparing these parameters with other temperature-dependent material parameters can help select circuit materials required for applications with high ambient operating temperatures (including meeting size, weight, power, and cost (SWaP-C) expectations).
Circuit materials are typically characterized at room temperature (RT) around +25°C, but the circuits they form may experience much higher operating temperatures. Due to the small size of mmWave circuits, increased operating temperature will lead to changes in mechanical and electrical performance. Increasingly, mmWave circuits will be installed in automotive radar and safety systems and fifth-generation (5G) cellular wireless communication networks, and the ultimate performance of these mmWave circuits, including thermal performance, will depend on the selection and consideration of circuit materials.
Since high temperatures can cause changes in the electrical and mechanical properties of circuit materials, there are a variety of parameters used to describe the effects of high temperatures on circuit materials. The coefficient of thermal expansion (CTE) can be used to describe the change in physical dimensions caused by temperature changes, while thermal conductivity is used to describe the heat flow characteristics of circuit materials.
Other temperature-dependent circuit material parameters are based on data from measurements of key material parameters such as dielectric constant (Dk) and dissipation factor (Df) as a function of temperature to infer how circuit materials and their circuits will change at high temperatures. Dk refers to the electromagnetic (EM) energy stored in two conductors equidistant in a vacuum and is commonly used in computer modeling and predicting the impedance of transmission lines and other circuit structures. Depending on the circuit material, Dk can change significantly at elevated operating temperatures, so it is important to understand how a circuit material’s Dk is affected by temperature. The temperature coefficient of dielectric constant (TCDk) has become a common circuit material parameter that provides insight into how a material’s Dk responds to elevated temperatures.
Ideally, no change in Dk (ΔDk) or ΔDk = 0 is the goal for most high-frequency circuit materials, although this is rarely achieved. This ideal material would have a TCDk of 0 ppm/°C, meaning that Dk would not change with temperature. In reality, however, all dielectric materials used in circuits will have varying degrees of Dk variation. More realistically, a TCDk of 50 ppm/°C or less is considered low and achievable, meaning that the circuit material has a very low change in Dk with temperature or a very low ΔDk value.
The dielectric loss or Df of a circuit material also changes as the temperature increases, and the temperature-dependent material parameter, the temperature coefficient of loss factor (TCDf), can be used to track these changes at specific temperatures and frequencies. The ideal TCDf value should be 0 ppm/°C with minimal variation over the operating temperature range.
Since it is difficult to accurately measure TCDf as it changes with temperature, insertion loss is a circuit parameter that more fully reflects the electromagnetic energy loss of a passive circuit on a specific circuit material. The temperature coefficient of insertion loss (TCIL) is also a temperature parameter that characterizes how the insertion loss of a circuit changes due to changes in operating temperature.
1. Test methods
By installing the test circuit on a heating device to control the operating temperature of the circuit, the temperature parameters of the circuit such as TCDk and TCIL can be accurately measured. The microstrip line differential phase length method is a method to accurately measure such temperature characteristics at millimeter wave frequencies (such as 77 GHz). During the test, the phase information of the microstrip transmission line can be measured under different conditions such as room temperature, +65°C and +125°C.
During the test, the different temperature environments are precisely controlled by devices such as thermal imagers and temperature sensors. The parameters of the microstrip lines tested are the same except for the length; such as the dielectric material, substrate thickness, and copper foil thickness. When the temperature of the circuit changes, the phase of the transmission line will change. Under millimeter wave frequency conditions, substrate materials such as 5 mil thickness and 1/2 oz can be selected to compare parameters such as TCDk and TCIL of different materials.
By testing the amplitude and phase data of the microstrip circuit at 77 GHz frequency band and different operating temperatures, the corresponding TCDk and TCIL data can be obtained. The phase information can be used to calculate the TCDk of the material, while the amplitude information can be used to obtain the TCIL performance of the material.
2. DK changes with temperature
To better understand the temperature performance of Rogers RO3003G2™ laminates, the microstrip line differential phase length method can be used to characterize the circuit TCDk and TCIL characteristics. RO3003G2 material is a circuit laminate based on polytetrafluoroethylene (PTFE) and ceramic spherical microparticle fillers, ensuring Dk consistency in all directions. In particular, performance optimization for millimeter wave bands, such as 77/79 GHz for automotive millimeter wave radars, further reduces material losses and ensures minimal Dk variation characteristics at high temperatures.
As mentioned above, the circuit characteristics of the circuit based on the 5 mil thick laminate were tested at different temperature conditions such as room temperature, +65°C and +125°C using the microstrip line differential phase length method, and the temperature-dependent TCDk and ΔDk, as well as TCIL and ΔIL obtained were compared. At the same time, the TCDk and TCIL of the 5 mil thick competitive material based on the polyphenylene ether (PPE) resin system were obtained using the same test conditions and methods. The temperature characteristics of the 5 mil RO3003G2 at 77 GHz were compared with the performance of the competitive material.
When using the microstrip differential phase length method to determine Dk at 77 GHz, the Dk value through the thickness (z-axis) of a 5 mil RO3003G2 laminate was 3.07. When the Dk was tested at 10 GHz using the fixed stripline resonator method, the Dk value along the z-axis was 3.00. Although both methods were performed at room temperature, it is not known how the Dk of a material changes when the circuit is subjected to higher operating temperatures. Using the microstrip differential phase length method, the Dk and frequency response of a material such as RO3003G2 laminate can be obtained over a wider operating frequency range and at room temperature. Extending this test, if the temperature is increased (+65° and +125°C) and the measurement is repeated, the TCDk can be determined and a better understanding of how changes in operating temperature affect the performance of the circuit of this material can be obtained.
As shown in Figure 1, the Dk of RO3003G2 laminate varies with frequency at various operating temperatures. First, the Dk of high frequency PCB materials decreases with increasing frequency; second, the Dk changes with increasing operating temperature. The TCDk of RO3003G2 laminate is -35 ppm/°C, and a series of measurements at different temperatures using the microstrip differential phase length method show that the Dk of the material decreases with increasing temperature, consistent with the negative sign. At 77 GHz, the ΔDk or Dk change of RO3003G2 material from room temperature to +125°C is approximately -0.010, and the phase angle change (Δϕ) is 6 degrees over a 1 inch (25.4mm) long microstrip transmission line.
This variation in Dk with temperature can cause undesirable performance changes in circuits at millimeter-wave frequencies, especially when the phase angle of high-frequency PCB transmission lines is designed to remain constant at all operating temperatures, and millimeter-wave signal magnitudes are assumed to remain constant in RF signals, transceivers, and other electronic systems. Although ΔDk is 0.010 at 77 GHz, especially when compared to millimeter-wave frequencies with other circuit materials, performance under different temperature conditions can be better understood.
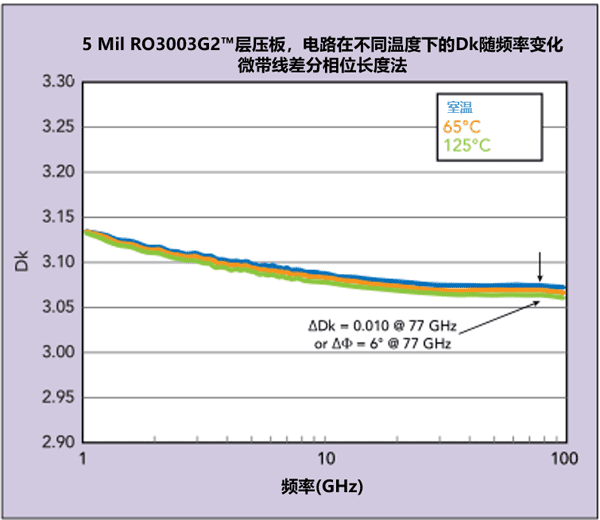
Figure 1. Measurement results show that the ΔDk of RO3003G2 circuit laminate at different temperatures at 77 GHz is very small.
For comparison purposes, a commercial high-frequency circuit material with a thickness of 5 mil currently on the market was tested and compared. The material data sheet indicates that the Dk in the z-axis direction at 10 GHz is 3.0 and the Df is 0.002. The microstrip line differential phase length method is used to measure the phase difference of transmission lines of different lengths on the laminate at 77 GHz, thereby evaluating the performance at millimeter-wave frequencies and the change in Dk at room temperature, +65°C, and +125°C. After testing, the ΔDk of the material at 77 GHz was 0.031 (Figure 2), which is three times as high as the (0.010) of the RO3003G2 laminate material. The change in the ΔDk value will also cause a very obvious change in the phase angle, and millimeter-wave radar applications usually focus on keeping the phase angle as consistent as possible.
As shown in the Dk vs. frequency graph, the Dk of this material changes relatively little at lower frequencies, with Dk values greater than 3.10. However, in the millimeter-wave frequency range, the change in Dk with temperature increases, indicating that the effect of temperature on Dk is very significant. As the operating temperature increases to a higher +125°C, the Dk at 77 GHz drops significantly from 3.045 to 3.010, a change of 0.031.
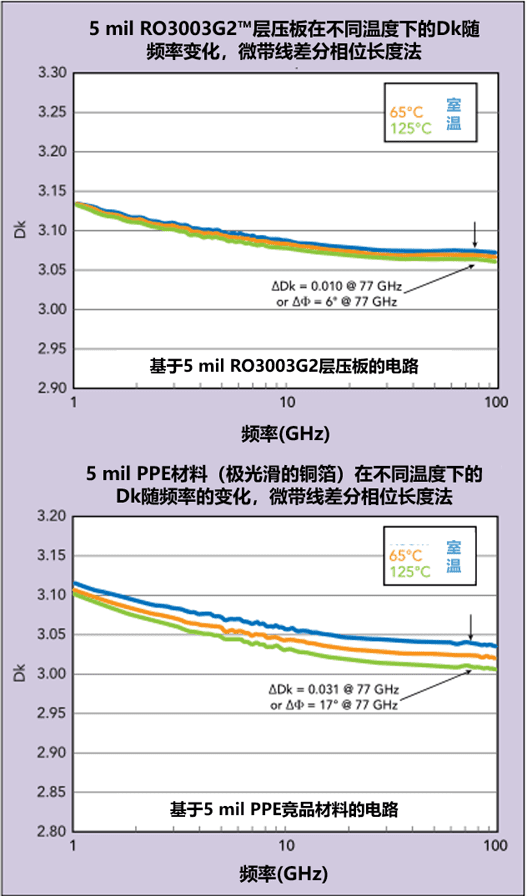
▲ Figure 2 The above chart shows the impact of different operating temperatures on the Dk of different high-frequency circuit materials at millimeter-wave frequencies.
3. Changes in loss with temperature
For circuit material loss variations with temperature, in addition to TCDf, the TCIL characteristic can provide a method to understand the overall loss performance of the circuit under different temperature conditions. TCDf is a material parameter that describes the trend of the material dielectric loss performance with temperature; TCIL is a circuit parameter that is used to describe the expected signal power loss of the circuit on the relevant circuit material. For active or passive circuits operating at millimeter-wave frequencies and in a wider temperature range, TCIL is also a method to evaluate the actual signal loss of components such as attenuators and filters used in millimeter-wave circuits or systems.
In order to evaluate the performance change of RO3003G2 circuit material in millimeter wave circuits at elevated temperatures , TCIL characteristics were tested in addition to TCDk. The test sample was the same circuit sample as previously tested, with 0.5oz standard HVLP ED copper foil and 5mil material thickness. It is well known that the insertion loss of all passive microwave/millimeter wave PCB transmission lines increases with frequency. As shown in Figure 3, the loss of the microstrip line circuit of RO3003G2 is also the same, with the circuit insertion loss at 77 GHz being approximately 1.3 dB/inch. At different operating temperatures of room temperature, +65°C, and +125°C, the insertion loss curves of RO3003G2 almost overlap, and the response to frequency is almost the same, with no significant change with temperature. It can be seen that the change in insertion loss or ΔIL at 77 GHz is only 0.008 dB/inch. Therefore, the loss of all passive circuits and transmission lines based on RO3003G2 material is less than 1.5 dB/inch at 77 GHz over a wide operating temperature range.
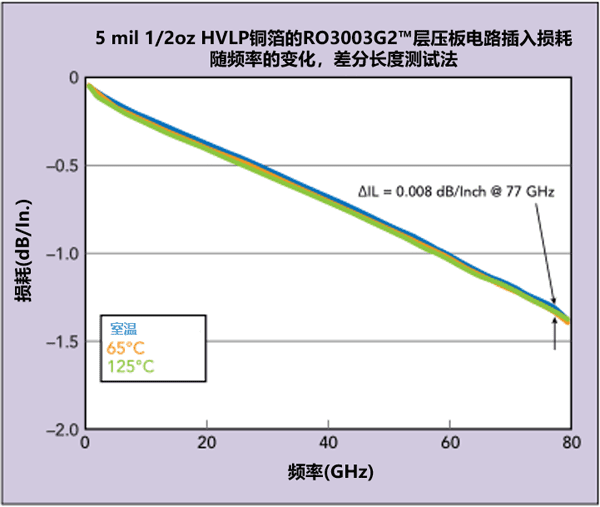
▲Figure 3 Insertion loss variation ΔIL of RO3003G2 circuit laminate based on PTFE at different temperatures
The evaluation of the loss of the PPE-based competitor’s high-frequency PCB materials over temperature was also tested using exactly the same method, and the insertion loss change ΔIL characteristics at different temperatures were tested. The design of the test circuit is also exactly the same, using 0.5oz ultra-smooth copper foil and 5 mil thickness of the competitor’s circuit material, and the microstrip line differential phase length test method. As shown in Figure 4, its insertion loss change over temperature is completely different from that of the RO3003G2 material, especially at 77 GHz. At 77 GHz, the insertion loss change ΔIL based on the PPE circuit material reaches 0.281 dB/inch. This means that the insertion loss of the 77 GHz radar millimeter wave circuit using this material will increase from 1.47 dB/inch at room temperature to 1.75 dB/inch at +125°C, with a loss change of more than 20%, which has a significant impact on the performance of the radar antenna and the radar detection distance.
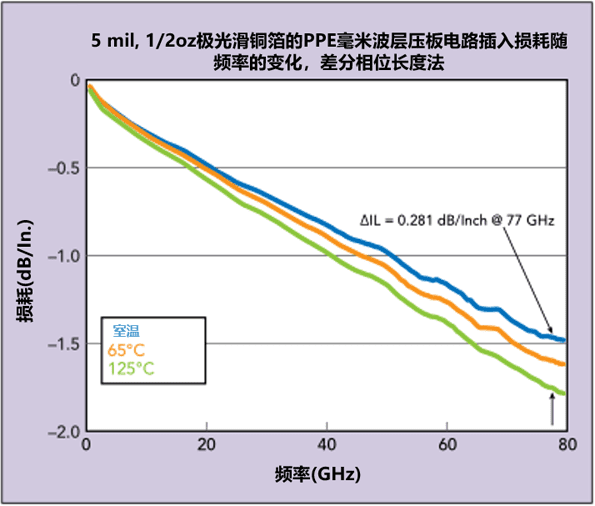
▲Figure 4 The insertion loss of circuit laminates based on PPE resin systems changes significantly with increasing temperature.
In addition to automotive radar sensors, the application of industrial sensors is increasingly dependent on the 77 GHz band and the 60~80 GHz band, so TCDk and TCIL are also very important for these bands. In order to better understand the frequency deviation that may occur in antennas at 77 GHz, a 1 × 8 series-fed microstrip patch antenna (as shown in Figure 5) was designed as a test sample to compare the frequency drift of different circuit materials at 77 GHz with temperature changes.
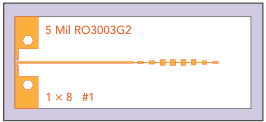
Figure 5 This is the antenna test circuit. A 77 GHz 1 × 8 series-fed microstrip patch antenna was designed on four different 5-mil-thick circuit materials to compare the antenna frequency offset caused by temperature changes.
The test process of using microstrip patch antenna is exactly the same as that of the previous microstrip line differential phase length method, except that the circuit under test uses patch antenna instead of microstrip transmission line. Patch antennas of exactly the same length are designed on 5 mil RO3003G2 and 5 mil CLTE-MW™ laminates, and two other different 5 mil PPE laminates (i.e., PPE #1 and PPE #2). The designed patch antenna is centered at 76.90 GHz with a bandwidth of about 1.5 GHz, and a quarter-wave impedance transformer is used for impedance matching of the antenna. The S parameters of the patch antennas on these four materials are measured according to the transmission line circuit temperature range discussed above. As shown in Table 1, the frequency deviation of the two antennas based on 5 mil PPE materials with temperature changes is significantly higher than that of 5 mil RO3003G2 and 5 mil CLTE-MW laminate materials. This also indicates that using these materials in an operating environment where the temperature changes may cause performance differences.
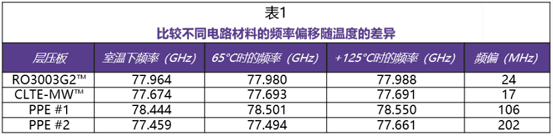
At millimeter-wave frequencies, where the transmitted signal power is limited, low loss characteristics are very important for circuits. Circuit materials with small insertion loss variations ΔIL at elevated temperatures are particularly important for applications such as automotive anti-collision radar. We know that automotive radar sensor systems have very small millimeter-wave signal loss variations and good performance consistency even in very harsh temperature environments, which can give system integrators (and automakers) the confidence that their radar sensor systems can operate reliably and normally within these temperature ranges.
Summarize
Changes in operating temperature environments are not always predictable, but they can be anticipated, especially for electronic applications that are exposed to outdoor temperatures and heat sources. Starting from the system’s circuit board, temperature effects can cause costly changes in circuit performance. A series of measurement techniques can help obtain and evaluate some material properties under elevated temperature conditions, such as parameters such as TCDk and TCIL, so that appropriate and high-performing circuit materials can be selected for key components.