Key Points in Automotive Radar Design from an RF Technology Perspective
Automotive radar technology is on the rise. Whether it is for current blind spot detection systems or for developing autonomous driving control, high performance, high reliability, compact size, and low cost are indispensable factors and motivations for the continuous development and improvement of core automotive radar technologies.
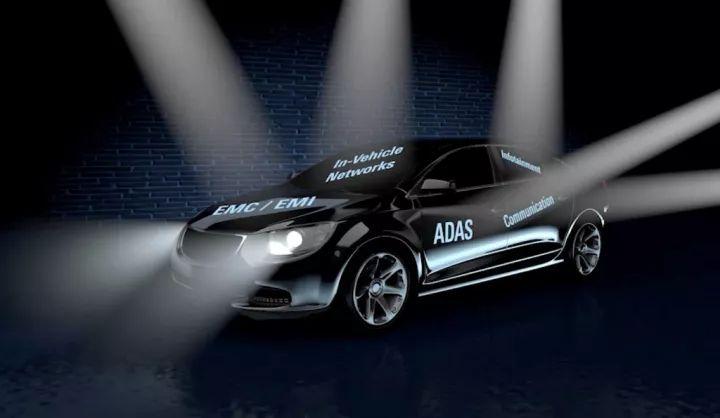
Automotive radar is an essential component of driver assistance systems. It not only provides a comfortable driving environment that reduces driver stress but also lays the necessary foundation for significantly improving road traffic safety. From designing and optimizing automotive radar to mass production and installation, various testing and measurement methods are employed. This article discusses several key design points of automotive radar from the perspective of RF (radio frequency) measurement technology.
Linearity of Radar Waveforms
Similar to other radar technologies, automotive radar relies on receiving the reflected signals from target objects and further analyzing the multiple correlations in time, frequency, and phase between the received signal and the original transmitted signal. This analysis helps determine the relative speed and spatial position of the target object in relation to the radar.
One of the core technologies of automotive radar is radar waveform design. Linear frequency modulated continuous wave (LFMCW, often referred to as FMCW) is a commonly used radar waveform. The stability and linearity of the transmitted signal (i.e., waveform) directly affect the radar’s ability to identify target objects. Since automotive radars typically operate in the millimeter-wave frequency band, the inherent nonlinear characteristics of various materials and components used will be reflected in the final transmitted and received signals, thereby interfering with signal analysis algorithms.
Automotive radar uses the frequency difference and phase difference between the transmitted and received signals to determine the speed and position of multiple target objects. When the linearity of the entire radar system, especially the transmitter part, exhibits non-ideal characteristics, the results of the frequency and phase difference calculations will become ambiguous, causing the radar system to incorrectly identify target objects and leading to significant errors. This is something that must be absolutely avoided for future autonomous driving control technology.
To minimize the error rate as much as possible, it is necessary to maximize the linearity of the transmitted signal and ensure the stability of this linearity through measurement. Due to the stringent requirements on signal quality, linearity measurements are often performed using high-end instrumentation to reduce measurement errors. Current high-end instruments can analyze signals with bandwidths exceeding 1 GHz to ensure comprehensive measurement of radar signals.
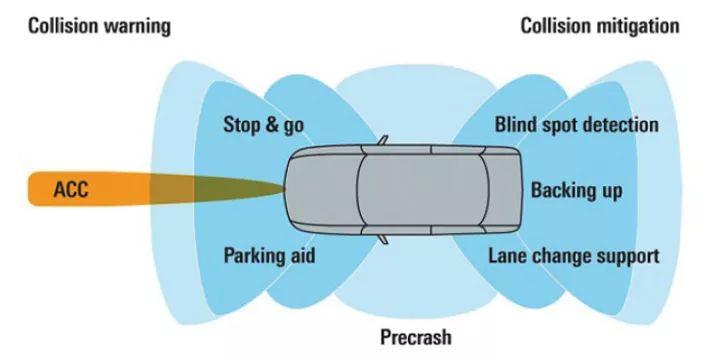
Automotive radar is rapidly expanding, providing more driver assistance and greater safety for vehicles.
Linearity of Radar Waveforms
In automotive radar applications, both transmitting and receiving antennas typically use phased arrays. Depending on the overall design requirements, linear arrays or planar arrays can be employed.
It is well known that the main parameters of array antennas (e.g., main lobe direction and width, side lobe suppression, null positions, etc.) can be calculated using simple mathematical formulas. However, the applicability of these calculations is conditional, specifically when the mutual coupling and influence between any two elements in the array are negligible. One method to meet this condition is to increase the relative distance between array elements. However, this method results in an increase in the size of the end product.
If calculations alone are insufficient for effective and accurate array element design, measurement becomes an important tool in the optimization process, supplemented by appropriate computer software to facilitate big data computations. The optimization of array antennas usually involves the following steps:
- Design of the radiation pattern of the basic array element
- Evaluation of mutual coupling between array elements
- Design of the radiation pattern of the antenna array
- Design of the array feeding system
- Integration design of the radar transceiver system
- Consideration of the impact of the vehicle bumper
Moreover, the overall design scheme of the radar system must also include requirements for safety and convenience, which directly or indirectly affect the antenna design, making it a highly interconnected process.
When designing the basic array elements, especially when evaluating mutual coupling between elements, the large number of elements in phased array antennas makes comprehensive, accurate, and rapid measurement crucial. To meet these requirements, multi-port vector network analyzers are indispensable.
Further considering safety and convenience requirements, the stability of the radiation pattern of automotive radar products in the same batch is of paramount importance. To achieve this goal, the measurement equipment for the radiation pattern must first provide good repeatability of measurement results, which is one of the challenges in automotive radar measurement technology.
In general, antenna radiation pattern measurements must be performed in the far field. The far field is a relative concept, defined according to commonly used antenna measurement theory, and is related to the size of the antenna under test and its operating frequency range. Specifically, the far field is proportional to the square of the maximum dimension of the antenna and inversely proportional to its operating wavelength.
For example, if an automotive radar product has a maximum dimension of 7.5 cm and operates at 24 GHz, the far field is approximately 0.9 meters away. However, if it operates at 77 GHz, the far field extends to around 3 meters. In typical mass production conditions, achieving such large distances is nearly impossible.
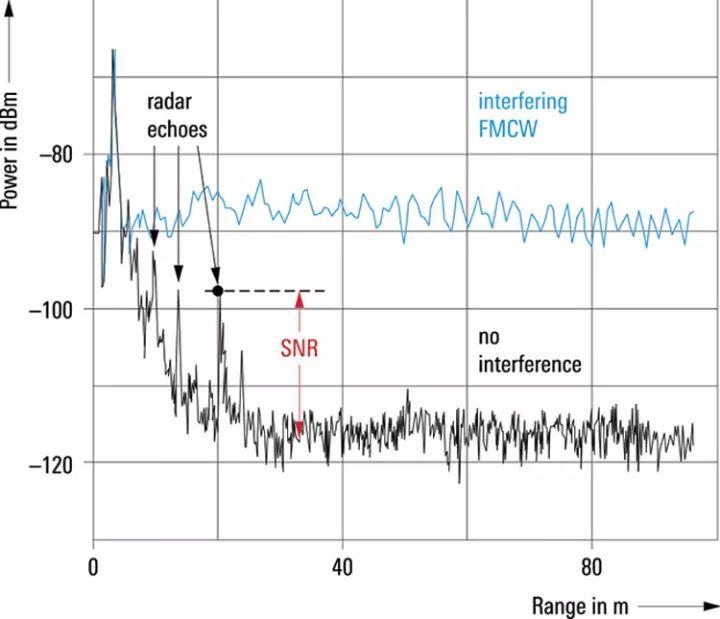
To overcome the limitations of the far-field definition, near-field data acquisition can be performed, and the collected data can then be converted into far-field results based on the near-field to far-field relationship. Although this near-field measurement method eliminates the distance range constraint, ensuring the accuracy of the measurement results requires highly complex measurement equipment and significantly increases the time needed for data acquisition. Furthermore, the data conversion process adds additional time, making the entire measurement process quite lengthy and difficult to meet the efficiency requirements of mass production.
The industry is currently exploring new methods to minimize these spatial and temporal constraints, thereby improving mass production capabilities within an appropriate distance range.
Interference and Anti-Interference
As with other RF products, automotive radar also faces challenges related to interference and anti-interference. Currently, the number of radars on a single vehicle is trending towards exceeding ten. Fortunately, through antenna design and proper adjustments during installation, mutual interference between radars on a single vehicle can be minimized. However, there are currently no design technical standards related to automotive radar, and the frequency range at which automotive radars operate does not require a license. This makes mutual interference between radars on different vehicles, especially those from different manufacturers, a particularly challenging issue.
Given the numerous unknown factors related to interference sources, during the design stage of anti-interference capabilities, it is essential to consider the worst-case scenarios, including those that initially seem unlikely, to accurately assess how the radar system might react unpredictably when subjected to interference.
As previously mentioned, automotive radar is primarily used to accurately and quickly determine the relative speed and spatial position between a target object and the radar. Errors in judgment can be categorized into two types: “false positives,” where the radar detects objects that aren’t there, and “misses,” where the radar fails to detect real objects. Both types of errors are critical for traffic safety and must be avoided in future autonomous driving control technology. If the relative distance is sufficiently long, there will be enough time to correct such errors.
Therefore, errors in judgment at relatively close distances are extremely dangerous. How should “relatively close distance” be assessed? For example, on urban roads with a typical speed limit of 60 km/hr, a distance of 15 to 20 meters can be considered “relatively close.” In such a distance, if a traffic anomaly occurs, the radar system must quickly and effectively engage the braking system to bring the moving vehicle to a stop within one second or less. The question is, can the radar system experience the aforementioned “false positives” or “misses” within this distance?
The answer is yes. In other words, due to interference with the receiving system, under certain conditions (including weather influences), the sensitivity of the automotive radar system to the reflected signals from target objects can dramatically decrease. While it can distinguish objects over 100 meters away, it might struggle to identify objects even 10 meters away under these conditions.
To ensure the anti-interference capability of automotive radars, standardized measurement methods are necessary. Although there are currently no regulatory measurement standards, the industry can reference other similar standards to conduct preliminary reliability measurements, thereby gaining time for early measurement. Once formal measurement methods are standardized, existing data can be used to appropriately adjust interference margins or enhance anti-interference capabilities based on regulations.
Current methods for detecting anti-interference capabilities primarily rely on software-configured scenarios. In an anechoic chamber, a signal source generates specific radar waveforms as interference sources, and a radar target simulator simulates specific target objects. This setup is used to evaluate the reduction in sensitivity of the tested radar to the reflected signals from target objects in the specific scenario, thereby estimating the anti-interference capability of the tested radar against the specific interference sources.
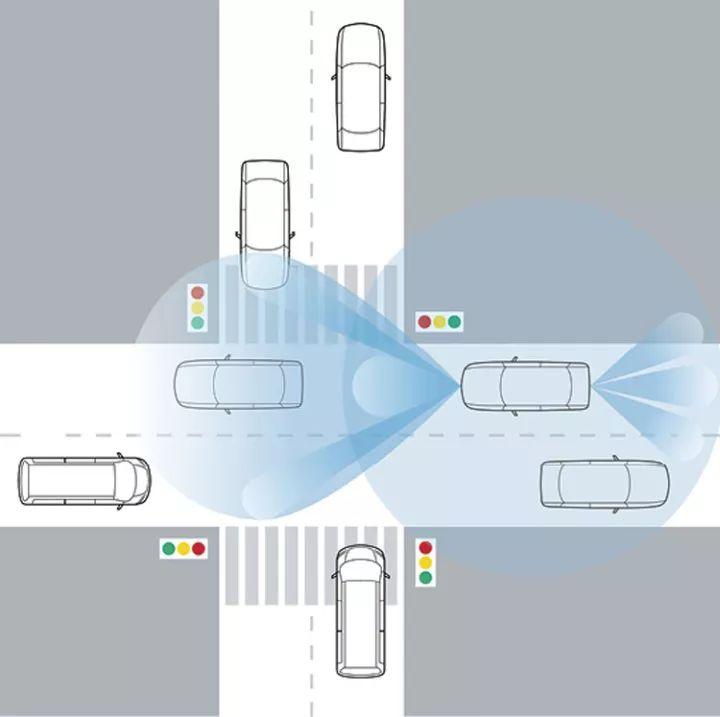
Different types of automotive radar sensors share a limited license-free spectrum, which can lead to mutual interference.
Conclusion
In summary, due to the critical importance of traffic safety, radar technology—one of the key technologies in autonomous driving control—still requires further development and improvement before autonomous driving becomes a part of everyday life. This includes the stability of radar waveform linearity, overcoming mass production technical bottlenecks, and enhancing anti-interference capabilities in various scenarios. Closely related to this is the RF measurement technology of automotive radars, which has already laid the necessary foundation for precise testing and future advanced measurement needs.